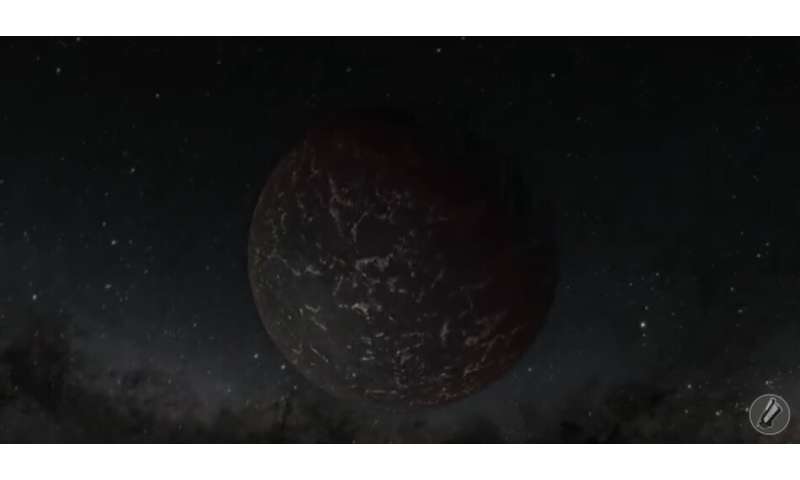
In the search for life beyond our galaxy, many scientists have their eyes turned toward orbs like Earth: rocky planets. So after the Transiting Exoplanet Survey Satellite (TESS) detected a rocky planet slightly larger than Earth last fall, a team of researchers launched a campaign to take additional images with the Spitzer Space Telescope, the only telescope currently in space that can directly detect a planet's infrared light. The telescope produced pictures smaller than 1 pixel—1/94 of an inch—like a speck of dust with which to make predictions about the planet's habitability.
Looking at several orbits of the planet allowed scientists to map the temperature of its surface and create models of its atmosphere—capabilities that scientists are only just starting to develop for rocky planets. Much of what researchers learn about exoplanets is based on what they know about the stars they circle.
"People say we only know a planet as well as we know the star, because we're basically inferring things based on what we're measuring about the star," said Laura Schaefer, an assistant professor of geological sciences at Stanford's School of Earth Energy & Environmental Sciences (Stanford Earth) and co-author on a study characterizing a planet that was published in Nature Aug. 19.
The team's analyses show that this planet, LHS 3844b, located 48.6 light-years away, is much hotter than Earth and may be covered in dark volcanic rock. It orbits a star smaller than the sun in just 11 hours. The star is an M dwarf—the most common and long-lived type of star that could therefore potentially host a high percentage of the galaxy's planets—and the rocky planet's atmosphere is the first orbiting an M dwarf to be characterized. Researchers found the planet has little to no atmosphere, and thus could not support life—an important finding for understanding atmospheres of similar rocky planets around M dwarfs.
Stanford News Service spoke with Schaefer to understand more about the findings and what they mean.
Why do scientists want to explore exoplanets?
Very broadly, it's to try to understand planet formation better. We understand in pretty good detail the planets in our own solar system, but that only gives us one snapshot of how planet formation works. By going out and finding planets around other stars, we have discovered many crazy new things that we didn't realize happened when planets formed. For example, we found one class of planets that nobody expected to exist, called hot Jupiters. These are actually the first kind of exoplanets that were discovered.
The other major goal with looking at exoplanets is to find another planet like Earth that might have life on it. I focus on the smaller rocky planets, not the big gas giants. The goal is eventually to find a planet in what we call the "habitable zone," which is a region of orbital space where liquid water might be stable on the surface of a planet like Earth.
In order to determine if a planet has life, we need to be able to measure its atmosphere and see if life has influenced it, as we know it has here on Earth, where our atmosphere of oxygen is produced by life. Before life was widespread on Earth, its atmosphere was very different. So we think if we can look at the atmospheres of planets in the habitable zone and determine what they're made of, then maybe we could say if those planets have life. This is a first baby step on the way to doing that.
How did the team map the temperature of a planet that's so far away?
By observing the planet at different points along its orbit, we see different fractions of the day side of the planet. If we're looking at the light from the star, we see a big dip when the planet is passing in front of the star, which we call the transit. As it goes behind the star, we see a smaller dip that we call the secondary eclipse. The amount of this dip gives us a constraint on the surface temperature of the planet. We can look also for variations in the stellar light that give us a temperature map with the day side and night side.
We can constrain the orbit pretty well; we know how close it is to its star and we know the star's brightness, so we know essentially how much light the planet is receiving from the star. We use models of the star's evolution to try to understand how much light that planet has received over its entire lifetime.
What did the data tell you about its atmosphere?
An atmosphere can take the heat from the star and move it around. If the planet doesn't have an atmosphere, then you would expect a big contrast between the day side and the night side. Two signatures of the atmosphere are a shift in the highest temperature point and a lower amplitude of this signature, which indicates that heat is being moved around. With this particular planet—one of the first rocky planets from which this kind of measurement has been able to be done—we found a large temperature contrast between the day and night side and no offset of that temperature point. That indicated that the atmosphere had to be really thin.
My contribution was to then determine if the atmosphere was stable by running models to look at how much atmosphere the planet could possibly lose for a range of parameters over the planet's lifetime. If the planet started off with about the same amount of gases, such as water and carbon dioxide, as Earth or even more than that, then it would have lost all of them over the course of its lifetime due to the star heating up the atmosphere and causing it to escape—that is one mechanism for atmosphere escape. We looked at another model that constrained the lower end of atmosphere the planet could have and determined these thin atmospheres are not stable on this planet.
Why do you focus your research on atmospheric escape models?
I started working on understanding early planetary atmospheres a number of years ago, before I even started grad school. To me, it's one of the most interesting problems because it's the early state of the planet that seems to really set how it evolves over its lifetime. That's really important for Earth, because we don't know a lot about its early history in the first half-billion years—but that's the time period when life started. So my perspective is you have to start at the beginning. And that actually means starting before the planet forms and trying to understand all the processes that go into making the planet and what sets the initial conditions that it eventually evolves from. By looking at these hot, rocky exoplanets, we get to test our understanding of these processes.
Explore further
Citation: Q&A: Scientist models exoplanet's atmosphere (2019, August 23) retrieved 23 August 2019 from https://phys.org/news/2019-08-qa-scientist-exoplanet-atmosphere.html
This document is subject to copyright. Apart from any fair dealing for the purpose of private study or research, no part may be reproduced without the written permission. The content is provided for information purposes only.
https://phys.org/news/2019-08-qa-scientist-exoplanet-atmosphere.html
2019-08-23 11:36:48Z
52780361339315
Tidak ada komentar:
Posting Komentar